Annals of Burns and Fire Disasters - vol. X
- n. 2 - June 1997
SKIN REGENERATION
AFTER HETEROLOGOUS EPIDERMAL SUBSTITUTE GRAFTING
Rouabhia M.
Laboratoire de
Recherche des Grands Brûlés/LOEX, Département de Chirurgie, Faculté de Médecine,
Université Laval, Québec (Qc), Canada
SUMMARY. Tissue
culture research has recently led to significant breakthroughs in several medical fields.
Autologous epidermal substitute transplantation for burn coverage and damaged skin
replacement is an example of such biotechnological progress. This approach has already
saved many lives. However, the main drawback to this treatment remains the time required
for patient keratinocyte growth before grafting. In order to overcome this problem, I have
devised a new cell culture method for rapid graftable epidermal substitute production.
These epidermal substitutes, composed of two keratinocyte populations, were grafted onto
an experimental model to assess their potential on generating functional skin. A hairless
mouse was chosen as a model for this heterologous epidermal substitute grafting. A
comparative study was carried out between the heterografts and the homogeneous implants.
Morphological, histological and immunohistochemical analyses showed that heterologous
epidermal substitutes led to successful graft take and functional skin regeneration.
Indeed, in situ analysis of the newly generated cutaneous tissue indicated good structural
organization, including the deposition of a continuous basement membrane and a well -v
ascularized neodennis. These data suggest the potential use of these heterologous
epidermal substitutes for clinical improvements in massive burn management.
Introduction
Burns injuries
affect millions of people in the world each year. An important number of these burned
patients require admission to a specialized burn unit. Over the past 40 years, great
progress has been accomplished in burn treatment leading to improved survival and
rehabilitation rates of patients. One of the major areas of progress has been the creation
of specialized burn units instead of burn care in general surgical departments where
patients were managed with inadequate and conservative treatments.
Progress consisted mainly of treating initial burn shock and pulmonary injuries, providing
suitable resuscitation, limiting infections, performing early excision of burn tissues,
and surgically reducing scars. In fact, the leading cause of death among burn shock
victims was inadequate fluid resuscitation in the first hours after injury.' In the 1960s
and 1970s, improvements in fluid resuscitation ruled out burn shock as a major cause of
mortality only to be replaced by wound sepsis as the leading cause of death among burn
patients. More recently, due to treatment of patients with topical and systemic
antibiotics, the principal cause of death is no longer wound sepsis but pulmonary sepsis
following inhalation injury.
Prior to 1950, the majority of massively and deeply (50%) burned patients died.' Since the
early 1990s, an equivalent mortality rate is seen only in patients with more than 95% of
total body burn surface.' The immediate priority when dealing with skin injury is to
control bleeding. Following this first medical assistance, prevention of burn wound sepsis
and wound coverage are the main priorities. The best way to permanently cover burns is to
transplant the patient's own cutaneous tissue, from an adjacent undamaged area of skin
that matches it closely in terms of texture, colour, and thickness. Generally, autografts
are meshed and expanded depending on the extent of the injury. Thickness and continuity of
the dermis provided in these autografts are known to be the major determinants of the
functional and cosmetic outcome of third-degree burns. In such patients, early closure of
burn wounds with autologous skin grafts is limited by the lack of adequate donor sites.
These donor sites and superficial burned surfaces, after spontaneous healing, are usually
reharvested two to three times. A delay of 2 to 3 weeks is required to allow these sites
to heal before reharvesting. However, harvesting deeply and frequently what was left as
healthy skin creates morbidity, including pain, infection, scarring, and, in some
patients, keloid formation on the donor site. This is particularly a problem in massive
burn injuries, where limited donor sites must be recropped, and in children and the
elderly, who have thin skin. This coverage process is also time consuming. To overcome
these limitations, alternative methods were developed. Since 1954, cadaveric allograft
skin has proved effective as a temporary covering for deep burns by decreasing evaporative
water loss through the wound and reducing the incidence of wound infection. Allograft
initially takes to the debrided wound bed, but is ultimately rejected as a consequence of
a specific immune response mounted against certain skin cells. Cells present in allograft
skin that contain or present major histocompatibility complex class I or 11 antigens
include keratinocytes, Langerhans cells (LC), endothelial cells, and some others. Despite
rejection, wounds covered with foreign skin, usually become free of infection and give
better cosmetic results. Allograft coverage stimulates epithelialization from the wound
margins and autologous remnants. In order to extend skin allograft survival, several
groups used immunosuppressive drugs, but since infection is a major risk of morbidity in
burn patients, their protocols did not gain much popularity. Rather than immunosuppressing
the patient, immunomodulation of the graft has been found to be a better and simpler
solution to overcome early rejection. Indeed, transplantation of allogeneic and xenogeneic
skin has several drawbacks such as problems of supply and high immunogenicity.
Consequently, the medical community must find alternatives to resuscitate and cover these
third-degree burned patients. Over the last decade, extensive efforts have been made which
have led to the development of in vitro cultured keratinocytes and graftable epidermal
substitute production .
This technique consists of isolation and culture of the patient keratinocytes obtained
from a CM2 biopsy of healthy skin . This culture procedure offers the possibility of
obtaining up to 10,000 times the initial biopsy surface .
The use of cultured autologous epidermal substitutes has steadily increased over the past
15 years and there are currently several laboratories throughout the world with tissue
culture facilities whose aim is to produce autologous epithelial grafts for use in a wide
variety of applications. For maximal clinical usage, rapid growth of autologous
keratinocytes has to be improved, because aging may reduce the proliferative potential of
these cells . Indeed, it has been reported that differences in the age of patients can
result in variations in the time needed to achieve confluence of cultures. This behaviour
is thought to be due to the declining growth potential of the basal keratinocytes. The
older the donor, the more the keratinocyte colony forming efficiency declines. However,
several other factors may affect the ability of the basal keratinocytes to grow in
culture.
Although epidermal substitute autografts have markedly advanced the management of
extensive burns and saved lives, one of their drawbacks is the time required (three weeks
and more) for the growth of keratinocytes in vitro. To reduce this time, some groups
hypothesize that allogeneic cultured epithelia might not be rejected because of the
elimination of immunologically active cells (lymphocytes and Langerhans cells) due to the
frequent changes of the culture medium. They observed a nonstimulation of T cells by the
allograft throughout the followup of the experiment and concluded that cultured epithelial
allografts were tolerated by the recipient. However, recent clinical and experimental
studies demonstrated that these allogeneic sheet grafts were rejected. Even when the
epidermal implants were depleted from immune cells, the rejection occurred. However,
before their elimination, cultured allografts transplanted onto deep partial skinthickness
burns induce a faster healing of the wound promoted by the residual resident keratinocytes
.2' Thus, the allograft may favour the proliferation and differentiation of spontaneously
regenerating epithelium.` The question is, are we able to generate functional skin by
grafting epidermal substitute containing allogeneic and syngeneic keratinocytes? If so,
these heterologous epidermal substitutes will have at least three major improvements: (i)
they are complete biological dressings; (ii) they can be produced as soon as possible on a
large scale; (iii) they allow permanent skin replacement. Clinically, these heterologous
epidermal substitutes will certainly reduce the patient's waiting time for damaged skin
cover and permanent replacement. To answer the question and evaluate the feasibility of
this co-culture method, heterologous epidermal substitutes were produced and grafted onto
immunodeficient mice (nu/nu), and the generated skin was analysed.
Materials and methods
New-born (1-3 day old) Balb/c and C3H/HeN inice were obtained from
Charles River Laboratories (SaintConstant, Quebec). Adult male athymic nu/nu mice (42 days
old) were also purchased from Charles River Laboratories of Canada and maintained under
sterile housing conditions. They were injected with ceftazidine 48 and 24 hours before
surgery in order to prevent infection. Other antibiotics (penicillin G and gentamicin, 100
IU/mI and 25 mg/ml, respectively, Sigma Diagnostics Canada, Toronto) were also added to
the sterile water for the same reason. The use of these antibiotics did not affect the
health and behaviour of the mice.
Epidermal cell isolation and culture
Keratinocyte suspensions were made using skin from new-bom mice (Balb/c and C3H/HeN)
according to a previously described method."," Briefly, new-bom mice (1-3 days
postpartum) were sacrificed by cervical dislocation following guidelines from the Canadian
Council on Animal Care, and the skin was removed with forceps. The epidermis was separated
from the dermis after incubation of the skin overnight at 4 'C in a 0.25%-20 pg/ml
solution of trypsin-DNase. The detached epidermal pieces were aseptically transferred to a
medium containing foetal calf serum (FCS) to inhibit residual enzyme activity, and
epidermal cells were then mechanically released. Cell suspensions were washed twice and
the pellets resuspended in 5 ml of culture medium and applied onto a Lympholyte-M gradient
(Cedarlane Laboratories Limited, Canada) and spun down at 300 x g for 30 min. 31,33
Epidermal cells at the interface were collected, washed twice, and resuspended in
supplemented culture medium.
Grqflable epidermal substitute production
After their isolation both types of keratinocyte popula~ tions (Balb/c and C311/HeN) were
plated separately or cocultured at the following ratios: 50% of Balb/e keratinocytes-50%
of C3H/HeN keratinocytes, or 75% of Balb/e keratinocytes-25% of C3H/HeN keratinocytes, or
25% of C311/HeN keratinocytes-75% of Balb/c keratinocytes. As control experiments,
homogeneous (100%) Balb/e and C3H/HeN keratinocyte cultures were respectively made up.
Cells were then incubated in a humidified atmosphere with 8% C02 at 37 'C until they
reached confluence. After 24 hrs of culture, epidermal growth factor (EGF) was added to
the medium. The medium was changed every 24 hrs until the epidermal cells reached
confluence.
The implant preparation and transplantation
Epidermal sheets were prepared for grafting when the primary cultures of keratinocytes had
reached confluence as previously described.` To graft these epidermal substitutes, athymic
nu/nu CD-1 male adult mice were anaesthetised with an intraperitoneal injection of
ketamine-xylazine at 0.05 ml/10 g weight. All the manipulations of the animals were done
with respect to the rules established by the Canadian Council on Animal Care. A 2-cm
incision was performed through the dorsal skin. The loose connective tissue under the
panniculus camosus was excised. A silicone chamber was implanted and heterologous
epidermal substitute was deposited on the muscle. As control experiments, homogeneous
(100%) Balb/c and C3H/HeN sheets were also transplanted. Five days later, the top of the
transplantation chambers was removed allowing macroscopic observations of the different
implants. The graft take was assessed clinically on days 14 and 30. The standardized
pictures produced were read with planimetric scales to obtain the percentage of take and
contraction of each epidermal substitute. The first group of grafted mice was sacrificed
on day 14 and the second on day 30 post-grafting for histological and immunohistochemical
analyses. Each experiment was performed four times and gave similar results.
In situ post-grafting analysis
The graft take was assessed on days 14 and 30, and photographed for macroscopic
evaluation. Standardized pictures were read with planimetric scales to obtain the
percentage of graft take and the area covered by each epidermal substitute .
Histological analyses: structural assessment
Fourteen and thirty days post-grafting, each implant was excised. Small biopsies were
then cut from these cutaneous tissue, fixed in HistoChoice Tissue Fixative* IX (Solon
Industrial Parkway, Ohio), and embedded in paraffin. Thereafter, 4-5 pm-thick sections
were stained with haematoxylin phloxine and saffron, and observed under a Nikon Optiphot
microscope as previously described.
Histochemical analyses: laminin and collagen-[V assessment. Intact biopsies were
harvested 14 and 30 days postgrafting from homografts (100% of the same keratinocyte
phenotype) and heterografts (50-50%, 25-75%), embedded in OCT compound (Miles, Elkhart,
IN), frozen in liquid nitrogen, and stored at -70 OC until used. Cryostat sections (4 pm)
were prepared from each biopsy. Sections were overlaid with a first antibody
(anti-laminin, anti-type IV collagen) for 45 min at room temperature in a 95% humidified
chamber. Sections were then rinsed extensively with phosphate buffer saline (PBS), and
overlaid with FITCconjugated (goat anti-mouse, goat anti-rat) for 45 min as above, in the
dark. Following further rinsing with PBS, sections were mounted in 30% glycerol-2%
glycine-PBS solution, overlaid with a coverslip, examined using a fluorescence microscope
(Nikon Optiphot), and photographed using Kodak Tmax 400 ASA film.
Tissue vascularization
To assess the vascularization of the newly generated cutaneous tissue, biopsy samples were
stained with antiCD31 monoclonal antibody. The CD31 antigen is an integral membrane
protein constitutively expressed on the surface of endothelial cells in a variety of
tissues. In skin, this protein was found around the vesselS.3' After labelling our implant
biopsies, they were examined using a fluorescence microscope and photographed.
Results
Morphological aspect of the implants.
The silicone biocompatible transplantation chamber provided adequate protection for each
implant. As shown in Fig. 1, fourteen and thirty days post-grafting, heterologous
implants showed morphological aspects comparable to the homogeneous implants. Indeed, it
was already possible to observe a good adhesion of epidermal substitutes as early as five
days post- transplantation, following the cap removal from the transplantation chamber. A
beautiful epidermis progressively cormfied in situ, without significant contraction of the
graft, was obtained (Fig. 1) 14 days post-grafting. However, some tension and
friction, induced on each implant by the backbones, delayed the evolution of the newly
generated cutaneous tissue. Thirty days post-transplantation, the graft take was over 90% (Figs.
1 d, e, f). Each
implant covered approximately the complete grafting bed. There was basically no
significant contraction of these epidermal substitutes 30 days after transplantation.
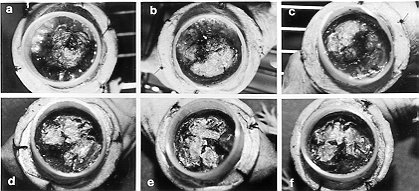
|
Fig. 1 - Photographs of the hairless mice grafted
with homogeneous or heterologous epidermal substitutes. Macroscopic aspect of the grafts
14 days (a, b, c) and 30 days (d, e, f) post-grafting. Three groups of mice were analysed:
(a, d) refer to homogeneous implants, (b, e) refer to 50%50% heterologous implants, (c, f)
refer to 25%-75% heterologous implants. Magnification, X 200 |
|
Histological analysis
Human epidermal evolution. Biopsies were taken on days 14 and 30 after transplantation
and stained using the haematoxylin phloxine and saffron method. This histological study
revealed a well-organized epidermis after heterologous epidermal substitute grafting (Fig.
2). Indeed, 14 days post-grafting, the newly generated cutaneous tissue after heterologous
epidermal substitute grafting was composed of several cell layers, including spinous and
granular regions (Fig. 2). This histological structure was similar to those obtained with
the homogeneous epidermal substitutes. In both grafts, the basal cells had retained their
cuboidal morphology and the formation of a stratum corneum, was in progress. The newly
generated epidermis of each implant category progressively increased in thickness leading
to the establishment of stratified epidermal layers at 30 days post-grafting (Figs. 2d, e,
f). Interestingly, the neodermis was well-organized 30 days post-grafting compared to the
14 day post-grafting.
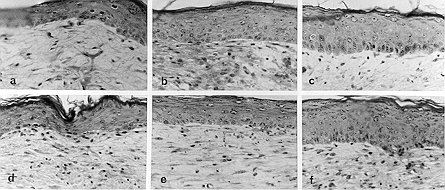
|
Fig.
2 - Structural organisation of the newly generated skin after homogencous and
heterologous epidermal substitute grafting. Biopsy specimens were harvested 14 and 30 days
postgrafting and stained using haematoxylin, phloxine and saffron staining method. Note
the histological organization of the epidermis with a continuous basal cell layers, the
stratum spinous, the stratum granular and the stratum corneum. We can also appreciate the
formation of the neodermis. Magnification, X 250. |
|
In situ basement membrane fbrmation. The
deposition of various basement membrane proteins was evaluated by immunofluoreseence. The
first molecule studied was laminin. Skin biopsies harvested 14 and 30 days post-grafting
from each epidermal substitute were stained with antilaminin and showed positive and
continuous basement membrane, labelling (Fig. 3). To confirm the functionality of
the newly generated cutaneous tissue through the basemerit membrane, type-IV collagen was
analysed. This analysis showed similar results (Fig. 4) to those obtained with
anti-laminin. The presence of these proteins in the newly generated cutaneous tissue
confirmed a well-structured epidermis with a dermo-epidermal junction. Heterologous and
homogeneous results were comparable.
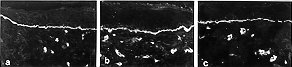 |
Fig. 3 - Laminin synthesis after homogeneous and
heterologous epidermal substitute grafting. Biopsy samples were taken 14 days
post-grafting and stained with anti-laminin monoclonal antibody. This staining showed
linear deposition of immunoreactants along the dermo-epidermal J . unction after 50-50%
(b) and 25-75% (c) heterologous implant grafting. Results were comparable to those
obtained after homogeneous (a) implant grafting. The same results were obtained 30 days
post-grafting. Magnification: X 200. |
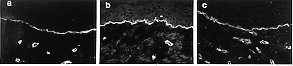 |
Fig. 4 - Type IV collagen synthesis after
homogeneous and heterologous epidermal substitute grafting. Biopsy samples were taken 14
days postgrafting and stained with anti-type 1V collagen monoclonal antibody. This
staining showed linear deposition of immunoreactants along the denno-epidermal junction
after 50-50% (b) and 25-75% (c) heterologous implant grafting. Results were comparable to
those obtained after homogeneous (a) implant grafting. The same results were obtained 30
days post-grafting. Magnification: X 200. |
|
Tissue vascularization
To assess the degree of vascularization of the newly generated cutaneous tissue, 14
days post-grafting homogeneous and heterologous biopsy samples were stained using an
anti-CD31 monoclonal antibody against platelet endothelial cell adhesion molecule. As
shown in Fig. 5, after both implants (homogeneous and heterologous epidermal substitutes),
the newly generated cutaneous tissues were well vascularized. The evaluation of vessel
number per sq. mm confirmed the important degree of vascularization in both implants (data
not shown). There was, however, no significant difference between the tissues regarding
their degree of vascularization. The same results were obtained 30 days after grafting
(data not shown).
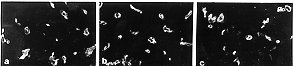
|
Fig. 5 - Tissue vascularization. Biopsy specimens
were taken 14 days after grafting and stained with anti-CD31 monoclonal antibody. This
staining showed immunoreactant deposition around vessels in the newly generated tissues
after 50-50% (b) and 25-75% (c) heterologous implant grafting. Results were comparable to
those obtained after homogeneous (a) implant grafting. Magnification: X 300. |
|
The presence of basement
membrane proteins (laminin and type IV collagen) and the good vascularization confirmed
that the heterologous epidermal substitutes allowed the generation of well-structured and
functional skin.
Discussion
Progress in the field of medical and
surgical resuscitation has led to an increase in the survival rate of patients affected
with extensive full-thickness burns. The modern treatment of deep burns consists of early
excision of burned tissue followed by immediate coverage with autograft, allowing
permanent skin replacement. However, in the case of extensive burns, healthy skin is
insufficient to cover the burned area even after maximal expansion. To overcome this
limitation, Green et al. developed a method to prepare in vitro graftable autologous
epithelia. This technique has now became fully integrated into the therapeutic strategy
for such patients. Clinical experience with cultured autologous epithelium was reported
first in 1981 and subsequently by many other teams in the world.
The advantage of this technique is that a
small biopsy of skin can provide enough epidermal sheets to cover the entire body surface
while avoiding the donor site wound. However, the initial enthusiasm generated by this
technique has been tempered because of serious limitations. The major drawbacks are the
time required for graftable sheet production, and the prohibitive cost when they are
industrially provided. However, they are of great help to surgeons who need to cover
extensively burned patients. This suggests that improvements to reduce at least one of
these major limitations will certainly help the medical community in its actions to save
these burn patients. For this purpose, I investigated in this study skin replacement using
heterologous implants (epidermal substitute containing two keratinocyte populations).
After production and transplantation of these heterologous epidermal substitutes similar
percentages of graft take (> 90%) were obtained as with the homogeneous grafts 14 days
post-grafting. Histological studies of the regenerated cutaneous tissue revealed a
well-organized epidermis containing basal and suprabasal cell layers. Immunofluorescent
staining of skin biopsy samples obtained from the newly generated cutaneous tissue showed
significant protein (laminin and type IV collagen) synthesis. There were, however, no
differences in the synthesis of either protein between both heterologous and homogeneous
implants. Moreover, anti-CD31 staining showed high vascularization of newly generated skin
after both types of epidermal substitute grafting, but there were no significant
differences between the grafts regarding their vascularization. Analyses were also
performed 30 days post-grafting and showed the same results (data not shown).
Consequently, the use of this co-culture method (mixture of allogeneic and autologous
keratinocyte populations) for large burn wound treatment could be a significant
therapeutic advance. We believe that the use of this new approach for human treatment may
diminish, by at least half, the previously described delay for epidermal culture and would
significantly reduce the cost of burn management during hospitalization.
On the basis of previous studies, it seems
clear that the use of cultured epidermis requires establishment of a rigorous
therapeutical strategy which requires the participation of all intervening personnel,
including cell culture biologists, who become essential partners. In plastic surgery,
keratinocyte sheets have been used to cover large skin defects after surgical excisions,
for giant congenital naevi, to cover the cavity after mastoidectomy, to graft the
separation site in combined twins, for tattoo removal, degloving injury, and for the
treatment of hypospadias.
Allogeneic keratinocytes have been reported to accelerate the treatment of extensive
second-degree burns and of graft donor sites. These allogeneic cells are later replaced by
autologous keratinocytes.
Autologous or allogeneic cultured epithelia are also used to stimulate the healing of
chronic wounds. The role of cultured epithelia for the treatment of junctional
epidermolysis bullosa has been described . They provide promising indications for oral
resurfacing in maxillofacial surgery. In conclusion, the production of bioengineered human
tissues has led to a fascinating diversity of medical applications, notably for permanent
burn wound coverage. Over the last decade, various skin substitutes have been introduced
for massive burn management, providing new approaches to reconstructive surgery.6l, The
heterologous epidermal substitute production method will certainly contribute to clinical
improvements of tissue and organ transplantation. This new culture technology could also
result in multiple applications and contributions to the development of the tissue
engineering field.
RESUME. Les
recherches récentes sur la culture des tissus ont porté de gros succès dans divers
champs médicaux. La transplantation des substituts épidermaux autologues pour la
couverture des brûlures et pour le substitution de la peau lésée est un example de ces
récents progrès biotechniques. Cette approche à déjà sauvé beaucoup de vies
humaines. Cependant, la méthode présente un handicap important, c'està-dire le temps
nécessaire pour la croissance des kératinocytes du patient avant la greffe. Pour
surmonter ce problème, j'ai créé une nouvelle méthode de culture des cellules pour la
production rapide de substituts épidermaux greffables. Ces substituts épidermaux,
composés de deux populations de kératinocytes, ont été greffés sur un modèle
expérimental pour évaluer leur capacité potentielle de générer une peau
fonctionnelle. Une souris sans poils a été sélectionnée comme modèle pour cette
greffe de substitut hétérologue. Une étude comparative a été effectuée entre les
hétérogreffes et les implants homogènes. Les analyses morphologiques, histologiques et
immunochirniques ont démontré que les substituts épidermaux hétérologues ont eu une
prise positive pour ce qui concerne la greffe et une bonne régénération fonctionnelle
de la peau. En effet, l'analyse in situ des nouveaux tissus cutanés a indiqué une bonne
organisation structurale, y compris la déposition d'une membrane basilaire continue et
bien vascularisée. Ces données proposent l'emploi potentiel de ces substituts
épidermaux hétérologues pour obtenir une amélioration clinique dans la gestion des
grandes brûlures.
BIBLIOGRAPHY
- Nguyen T.T., Gilpin D.A., Meyer N.A. et
al.: Current treatment of severely burned patients. Ann. Surg., 223:14-25, 1996.
- Monafo W.W.: Then and now: 50 years of burn
treatment. Burns, 18(S2): S7-SIO, 1992.
- Masellis M., Gunn S.W.A.: The management of
burns and fire disa- sters: perspectives 2000. Kluwer Academic Publishers, Lancaster, UK,
1995.
- Muller M.J., Herndon D.N.: The challenge of
burns. Lancet, 343: 22.216-20, 1994.
- Bull J.P., Fisher A.J.: A study of
mortality in a burn unit: a revised estimate. Ann. Surg., 139: 269-74, 1954.
- Herfidon D.N., Rutan R.L.: Comparison of
cultured epidermal auto graft and massive excision with serial autografting plus homograft
overlay. J. Burn Care Rehabil., 13: 154-7, 1992.
- Freeman A.E., Igel H.J., Waldman N.L.: A
new method for covering large surface area wounds with autografts. Arch. Surg., 108:
721-3, 1974.
- Fang C.-H., Alexander JW.: Wound
contraction following trans plantation of microskin autografts with overlaid skin
allograft in experimental animals. Burns, 16: 190-2, 1990.
- Green H., Kehinde 0., Thomas J.: Growth of
cultured human epidermal cells into multiple epithelia suitable for grafting. Proc. Natl.
Acad. Sci. USA, 76: 5665-8, 1979.
- Shirani K.Z., Vaughan G.M., Mason A.D. Jr
et al.: Update on current therapeutic approaches in burns. Shock, 5: 4-16, 1996,
- Linares H.A.: From wound to scar. Burns,
22:339-52, 1996.
- Jackson D.: Clinical study on the use of
skin homografts for burns.Br. J. Plast. Surg., 7: 26-43, 1954.
- Wong L.: The many uses of allograft skin.
Ostomy Wound Manage,41: 36-42, 1995.
- Hefton J.M., Madden MR., Finkelstein J.L.
et al.: Grafting of burn patients with allografts of cultured epidermis cells. Lancet, 2:
428-30,1983.
- Thivolet J., Faure M., Demidern A. et al.:
Long-term survival and mmunological tolerance of human epidermal allografts produced in
culture. Transplantation, 42: 274-80, 1986.
- Fame M., Mauduit G., Schmitt D. et al.:
Growth and differentiation of human epidermal cultures used as auto- and allografts in
humans.Br. J. Dermatol., 116:161-70, 1987.
- Wong L.: The many uses of allograft skin.
Ostomy Wound Manage, 41: 36-42, 1995.
- Burke J.F., May JW. Jr, Albright N. et al.:
Temporary skin transplantation and immunosupression for extensive burns. New Engl. J.Med.,
290: 269-71, 1974.
- Eldad A., Benmeir P., Weinberg A. et al.:
Cyclosporin A treatment failed to extend skin allograft survival in two burn patients.
Burns, 20: 262-4, 1994.
- Rue L.W. 111, Cioffi W.G. Jr, McManus W.F.
et al.: Wound closure and outcome in extensively burned patients treated with cultured
autologous keratinocytes. J. Trauma, 34: 662-8, 1993.
- Rouabhia M., Germain L., Bergeron J. et
al.: Allogeneic-syngeneic cultured epithelia. A successful therapeutic option for skin
regeneration. Transplantation, 59: 1229-35, 1995.
- Germain L., Rouabhia M., Guignard R. et
al.: Improvement of human keratinocyte isolation and culturing using tbermolysin. Burns,
19: 99-104, 1993.
- Rheinwald J.G., Green H.: Serial
cultivation of strains of human epidermal keratinocytes: the formation of keratinizing
colonies from single cells. Cell, 6: 331-44, 1975.
- Munster A.M., Weiner S.H., Spence R.J.:
Cultured epidermis for the coverage of massive burn wounds. Ann. Surg., 211: 676-80, 1990.
- Latarjet J., Grangolphe M., Hezez G. et
al.: The grafting of burns with cultured epidermis as autografts in man. Scand. J. Plast.
Reconstr. Surg. Hand Surg., 21: 241-4, 1987.
- Fisher J.C.: Skin, the ultimate solution
for the burn wound. N. Engl.J. Med., 311: 466-7, 1984.
- Aub6ck J., Irschick E., Romani N. et al.:
Rejection after a slightly prolonged survival time of Langerhans cell-free allogeneic
cultured epidermis used for wound coverage in humans. Transplantation, 45: 730-7, 1988.
- Rouabhia M., Germain L., Belanger F. et
al.: Cultured epithelium allografts: Langerhans cell and Thy-I+ deDdritic epidermal cell
depletion effects on allograft rejection. Transplantation, 259-64, 1993.
- De Luca M., Albanese E., Bondanza S. et
al.: Multicentre experience in the treatment of burns with autologous and allogeneic
cultured epithelium, fresh or preserved in a frozen state.Burns, 15: 303-9, 1989.
- Oliver A.M., Kaawach W, Mithoff E.W. et
al.: The differentiation and proliferation of newly formed epidermis on wounds treated
with epithelial allografts. Br. J. Dermatol., 125: 147-54,1991.
- Rouabhia M., Germain L., B61anger F. et
al.: Optimization of murine keratinocyte culture for the production of graftable epidermal
sheets. J. Dermatol., 19: 325-34, 1992.
- Rouabhia M.: In vitro production and
transplantation of immunologically active skin equivalents. Lab. Invest., 75: 503-17,
1996.
- Rouabhia M., Jobin N., Doucet R. Jr et al.:
CD36+-dendritic epidermal cells: a putative actor in the cutaneous immune system. Cell
Transplant, 3: 529-36, 1994.
- Rouabhia M.: Permanent skin replacement using chimeric
epithelial cultured sheets comprising xenogeneic and sygeneic keratincoytes.
Transplantation, 61: 1290-300, 1996.
- Xu W., Germain L., Goulet F. et al.: Permanent grafting of
living skin substitutes: surgical parameters to control for successful results. J. Burn
Care Rehabil., 17: 7-13, 1996.
- Vecchi A., Garlanda M.G., Lampugnani M. et al.: Monoclonal
antibodies specific for endothelial cells of mouse blood vessels. Their application in the
identification of adult and embryonic endothelium. Eur. J. Cell. Bio., 63: 247-54, 1994.
- Jackson R, Loughrey C.M., Lightbody J.H. et al.: Effect of
hemodialysis on total antioxidant capacity and serum antioxidants in patients with chronic
renal failure. Clin. Chem., 41: 1135-8, 1995
- Janzekovic Z.: A new concept in the early excision and
immediate grafting of burns. J. Trauma, 10: 1103-8, 1970.
- O'Connor N.E., Mulliken J.B., Banks-Schlegel S. et at:
Grafting of burns with cultured epithelium prepared from autologous epidermal cells.
Lancet, 1: 75-8, 1981.
- Gallico G.G., O'Connor N.E., Compton C.C. et al.: Permanent
coverage of large burn wounds with autologous cultured human epithelium. N. Engl. J. Med.,
311: 448-51, 1984.
- Auger A.F.: The role of cultured autologous human
epithelium in large burn wound treatment. Transplantation/Implantation Today, 5:
21-6,1988.
- De Luca M., Bondanza S., Cancedda R. et al.: Permanent
coverage of full skin thickness burns with autologous cultured epidermis and
reepithelialization of partial skin thickness lesions induced by allogeneic cultured
epidermis: a multicentre study in the treatment of children. Burns, 18:16S-18S, 1992.
- Hemdon D.N., Rutan L.R.: Comparison of cultured epidermal
autograft and massive excision with serial autografting plus homograft overlay. J. Burn
Care Rehabil., 13: 154-7, 1992.
- 0Tompkins R.G., Hilton J.F., Burke J.F. et al.: Increased
survival after massive thermal injuries in adults: preliminary report using artificial
skin. Crit. Care Med., 17: 734-40, 1989.
- Sheridan R.L., Hegarty M., Tompkins R.G. et al.: Artificial
skin in massive burns - results to ten years. Eur. J. Plast. Surg., 17: 91-3, 1994.
- Teepe R.G.C., Ponec M., Kreis R.W. et al.: Improved
grafting method for treatment of burns with autologous cultured human epithelium (letter).
Lancet, i: 385, 1986.
- Donati L., Magliacani G., Bormioli M. et al.: Clinical
experiences with keratinocyte grafts. Burns, 18: 19S-25S, 1992.
- Barillo D.J., Naugle M.E., Farrell K.: Preliminary
experience with cultured epidermal autograft in a Community Hospital Burn Unit. J. Burn
Care Rehabil., 13: 158-65, 1992.
- Woodley D.T.: Covering wounds with cultured keratinocytes.
JAMA, 262: 2140-1, 1989.
- Gallico G., O'Connor N.E., Compton C. et al.: Cultured
epithelial auto grafts for giant congenital naevi. Plast. Reconstr. Surg., 84: 1-9, 1989.
- PremachandraD.J., Woodward B.M., Milton C.M. et al.:
Treatment of post-operative otorrhoea by grafting of mastoid cavities with cultured
autologous epidermal cells. Lancet, i: 365-7, 1990.
- Higgins C.R., Navsaria H.A., Stringer M. et al.: Use of
two-stage keratinocyte-dernial grafting to treat the separation site in conjoined twins.
J. R. Soc. Med., 87: 108-9, 1994.
- Abbes M., Bourgeon Y., Regnier M. et al.: Notre expérience
dans l'utilisation des cultures de cellules épidermiques en chirurgie réparatrice. Ann.
Chir. Plast. Esthet., 35: 31-8, 1990.
- Clarke J.A.: Cultured skin for burn injury. Lancet, 2: 809,
1986.
- Romagnoli G., DeLuca M., Faranda F. et al.: Treatment of
posterior hypospadias by the autologous graft of cultured urethral epithelium. N. Engl. J.
Med., 323: 527-30, 1990.
- De Luca M., Bondanza S., Cancedda R. et al.: Permanent
coverage of full skin thickness burns with autologous cultured epidermis and
re-epithelialization of partial skin thickness lesions induced by allogeneic cultured
epidermis: a multicentre study in the treatment of children. Burns, 18: 16S-18S, 1992.
- Phillips T.J., Gilchrest B.A.: Clinical applications of
cultured epithelium. Epith. Cell. Biol., 1: 39-46, 1992.
- Myers S., Navsaria H., Sanders R. et al.: Transplantation
of keratiocytes in the treatment of wounds. Am. J. Surg., 170: 75-83, 1995.
- Van Der Merwe A.E., Mattheyse F.J., Bedford M. et al.:
Allografted keratinocytes used to accelerate the treatment of burn wounds are replaced by
recipient cells. Burns, 16: 193-7, 1990.
- Leigh I.M., Purkis P.E.: Culture grafted leg ulcers. Clin.
Exp. Dennatol., 11: 650-2, 1986.
- Phillips T.J., Gilchrest B.A.: Cultured allogeneic
keratinocyte grafts in the management of wound healing. Dermatol. Surg. Oncol., 15:
1169-76, 1989.
- Carter D.M., Lin AX, Varghese M.C. et al.: Treatment of
junctional epidermolysis bullosa with epidermal autografts. J. Am. Acad. Dennatol., 17:
246-50,1987.
- Schofield O.M.V., Casella J.P., Navsaria H.A. et al.:
Cultured keratinocyte allografts in dystrophic epidermolysis bullosa: preliminary
observations. Br. J. Dermatol., 123: 66A, 1990.
- McGrath J.A., Schofield M.V, Yamamoto A.I. et al.: Cultured
keratinocyte allografts and wound healing in severe recessive dystrophic epidermolysis
bullosa. J. Am. Acad. Dermatol., 29: 407-19, 1993.
- Langdon J., Williams D.M., Navsaria H.A. et al.: Autologous
keratinocyte grafting: a new technique for intra oral reconstruction. Br. Dent. J., 171:
87-90, 1991.
- Ueda M., Ebata K., Kaneda T.: In vitro fabrication of
bioartificial mucosa for the reconstruction of oral mucosa. Basic research and clinical
applications. Ann. Plast. Surg., 27: 540-9, 1991.
- Kitano J., Okada N.: Separation of the epidermal sheets by
dispase. Br. J. Dermatol., 108: 555-8, 1983.
- Rouabhia M. (ed.): Skin substitute production by tissue
engineering: clinical and fundamental applications. R. G. Landes Company, Biomedical
Publishers, Austin TX, USA, in press: 1997.
Boyce S.T., Ham R.C.: Calcium-regulated
differentiation of normal epidermal keratinocytes in chemically defined clonal culture and
serum-free serial culture. J. Invest. Dermatol., 81: 33S-40S, 1983.
|