Annals of Burns and Fire Disasters - vol. XII - n. 1 - March 1999
A NEW DELIVERY
SYSTEM OF ANTIBIOTICS IN THE TREATMENT OF BURN WOUNDS
Giannola L.I.,* De Caro V.,*
Adragna E.,* Giandalia G.,* Giannola G.,* D'Arpa N.,** Napoli B.,** D'Amelio L.M.,**
Genovese LM,** Lombardo C.,** Masellis M.**
*
Dipartimento di Chimica e Tecnologie Farmaceutiche, Università di Palermo, Palermo, ltaly
** Divisione di Chirurgia Plastica e Terapia delle Ustioni, A.R.N.A.S. Ospedale Civico e
Benfratelli *** G. Di Cristina - Maurizio Ascoli", Palermo
SUMMARY. The
development of a new antibiotic delivery system suitable for application on burn wounds is
described. The system was designed to be administered together with an implant of
epidermal cultured keratinocyte stem cells. The antibiotic was entrapped in a reservoir
compartment which was capable of releasing the drug at a controlled rate. The desired
delivery profile was determined in vitro using a general two-compartment linear
time-invariant model. The suitable rate for a release of the antibiotic in sufficient
amounts for a therapy of 5-6 days was obtained using a combined mechanism based on
diffusion through a membrane and decrease of drug movement properties in the vehicle. The
topical release system that is proposed limited the indiscriminate use of broad-spectrum
antibiotics, thus reducing the possible incidence of undesirable mulfiresistance.
Interruption of the system in the area after five days of treatment was easy as it caused
the patient no pain, no trauma to the zone, and no damage to the graft. Eight days after
application it was observed that the treatment of patients with cultured keratinocytes had
been successful and that the lesions had healed.
Introduction
It is well known that the burn,
compared with other forms of trauma, produces extensive skin barrier disruption together
with devitalization of tissues, which causes the formation of large raw areas. The burn
wound has a much higher incidence of infection than other forms of trauma, owing to
alteration of the cellular immune responses.' Cultured epidermal keratinocyte allografts
or autografts have been used as a biological dressing for burn wounds. Flexible natural or
synthetic thin films applied to the grafts may be used as barriers for the damaged skin
against dust and dirt. However, the contamination of the wound by virulent microflora is
often responsible for ineffectiveness of treatment. Infection remains the major problem in
the treatment of patients, leading to the indiscriminate use of broad-spectrum antibiotics
that transform burn wounds into sites of multiresistant virulent bacteria.
During the past decade a plethora of reports has appeared in the literature regarding
dermal and transdertnal delivery of drugs through intact skin with the aim of duplicating
the benefits of intravenous drug infusion while avoiding its hazards. An extensive study
has been made of the principles, mechanisms, and physicochemical parameters important in
the control of percutancous penetration of drugs as well the qualitative and quantitative
aspects in the prediction of absorption.
Although a considerable amount of research has been devoted to transdermal drug delivery
systems, very little work has been done on applications on skin following thermal injury.
The acceptability of a delivery system as a drug administration option can be dramatically
altered when burn wounds are involved.
Drugs can be released by dermal and transdermal delivery systems using different
mechanisms. The physical and chemical properties of both the drug and the system
components can be optimized to reach the desired release profile, although the skin's
barrier properties tend to normalize the amount of penetrating drug molecules.
For the topical application of drugs on damaged skin, when there is no barrier, primary
resistance to mass transfer depends on the vehicle in which the active ingredient is
dispersed. Drug discharge from the vehicle can be regulated by changing the movement
properties of the active ingredient in the vehicle itself, by varying the thickness of the
layers applied, or by choosing appropriate formulation components. The use of various
polymeric vehicles allows optimization of the drug liberation rate from conventional
pharmaceutical dosage forms. The selection of polymer and other components thus becomes
critical to the success of the formulation. The desired release rate and drug level can
also be achieved by using appropriate delivery systems. An excellent liberation rate can
also be achieved using films or membranes to match the diffusivity of the drug molecules
by selecting useful plasticizers or vehicles, or naturally occurring, sernisynthetic, or
synthetic polymers.
The present study reports on a re-evaluation of therapeutic practices and on the
development of a new antibiotic delivery system suitable for application in burn patients.
The system was designed to be administered after the implantation of epidermal cultured
keratinocyte stem cells and was capable of releasing antibiotics with ratecontrolled
characteristics. In this type of pre-programmed drug delivery system, the active
ingredient is entrapped in a reservoir compartment and covered by a rate-controlling
membrane having a specific permeability. The membrane controls the molecular diffusion of
drugs crossing the barrier and passing into the surrounding environment at a
pre-programmed rate. The resultant equation was C = 0.4159 V'-t~ 0.8649.
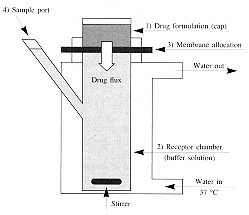
|
Fig.
1 - Diagramatic illustration of a Franz-like diffusion cell. |
|
Materials and methods
In vitro experiments: apparatus
The diffusion of antibiotics was determined using Franz-type diffusion cells (Fig. 1).
Each cell included four major elements:
- formulation chamber for the source of diffusing drug to be
tested (cap);
- receptor chamber for the simulated biological medium (body)
into which the drug was transferred;
- membrane allocation site, separating the donor from the
receptor compartment; and
- side arm for withdrawal of samples (sample port).
Accurately weighed quantities of
pharmaceutical fon-nulation were placed in the cap to apply the same thickness layer of
material in every cell. The receptor chamber of every cell was filled with 42 ml of
isotonic phosphate buffer solution (pH 7.4) used as a simulated receptor phase. The
temperature was maintained at 37 ± 0.5 'C by means of thermostatically controlled water
entering the lower port of the water jacket surrounding the body chamber and circulating
out through the upper port. All the cells had an exposed surface area of 10.7 CM2 . The
receptor solution was stirred by means of a magnetic follower rotating at 600 rpm, which
greatly increased mixing efficiency and reduced the tendency to form a stagnant boundary
layer next to the membrane surface. The agitation speed was established to maintain a
hydrodynamic condition such that the thickness of the diffusion boundary layer was at a
minimum; the primary convective flow below the drugreleasing surface was also minimized.
The degree of dispersal of coloration from pennanganate was used to make a qualitative
assessment of the stirring rate. At regular time intervals (30 min or I h) samples (I ml)
were removed from the centre of the receptor chamber through the sample port, using a long
needle and a syringe. To avoid saturation phenomena and to maintain the sink conditions,
the sample volume taken was replaced by fresh buffer solution, injected by syringe,
ensuring that no air was drawn into the compartment.
Drug permeation was monitored by analysis of the cumulative amount of antibiotic reaching
the receptor phase. For each experiment the percentage amount of drug transferred was
plotted as a function of time. Each experiment was repeated five times. The results are
expressed as the mean ± SEM. Reproducibility was within 3% of the mean. Fig. 2 shows
the cumulative amounts of vancomycin permeating from the formulation through VelodermO,
which functioned as a diffusion membrane and gave reproducible results. The residual drug
content in the formulation and the amount of drug released matched the original content,
confirming that no drug was entrapped in Veloderm@.
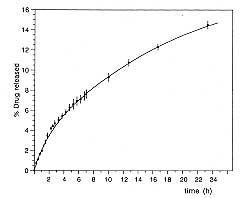
|
Fig.
2 - Percentage amount of vancomycin transferred into the acceptor
compartment versus time. |
|
UV spectrophotornetry
The detection of vancomyein was carried out by UV spectrophotometry with a Shimadzu
1601 UV-VIS instrument, using the appropriate blank and calibration curve at 280.4 mn. The
standard solutions had concentration ranges between 0.06 and 0. 15 mg/ml, which were the
same as those predicted in the samples from the cells. The calibration curve gave the
specific extinction coefficient (E,,, 1 cm = 0.034 at 280.4 nm) of vancomycin
hydrochloride with a correlation coefficient 0.9996.
Preparation of vancomycin gel-like
dispersion for in vitro experiments
Vancomycin hydrochloride (256 mg), corresponding to 250 mg of vancomycin, was
dissolved in 97 ml of a sterile, apyrogenic and isotonic phosphate buffered saline (pH
solution, 7.0). Three grams of hydroxyethyleellulose were added to the solution. The
resulting mass was sonicated in a Branson 5200 ultrasound bath until a homogeneous-like
gel dispersion was obtained.
All manufacturing procedures for pharmaceutical dispersion were performed in order to
ensure the product's sterility.
Keratinocyte in vitro culture
technique
The skin biopsies were harvested from burn patients, cleaned with 70% ethanol, and
processed according to Rheinwald and Green's keratinocyte isolation method.' Skin biopsies
were trypsinized overnight at 4 'C in a 0.05% trypsin/0.02% EDTA solution. The cells were
isolated from the epidermal/dermal junction and plated onto a feeder monolayer of lethally
irradiated 3T3 cells. The medium was made up of three parts Dulbecco's modified with
Eagle's medium plus one part Ham's F12 medium. The following were added to the mixture:
cholera toxin (0.1 nM), hydrocortisone (0.4 pg/ml), triiodo-l-thyronine (20 pM), insulin
(5 pg/ml), transferrin (5 pg/ml), fungizone (250 pg/ml), penicillin (1000 IU/ml),
streptomycin (1000 p g/ml), and 10% foetal calf serum. Epidermal growth factor (10 ng/ml)
was added with the first medium change. Cells were cultured at 37 'C in a 5% CO
atmosphere. The medium was changed every two days.
Subconfluent primary cultures were trypsinized and cells were plated in a secondary
culture. The confluent secondary cultures were detached from the surface vessel with the
neutral protease Dispasell, washed in serum-free medium, and placed on sterile Veloderm@.
Preparation of wound bed
All patients included in the study
presented deep thirddegree flame burns. The wounds were treated by surgical intervention
and autoskin grafting, remaining uncovered after treatment.
The preparation of the wound bed for the application of the antibiotic delivery system was
of decisive importance for the outcome of the whole procedure. The wound bed had to be
clean, free from necrotic tissue residue, and with minimum contamination. Bacteriological
surveillance was performed by monitoring the damaged zone at every medication in order to
observe any signs of sepsis. Wound care included the application of antibiotic cleanser
solutions as topical agents. The cleanser was prepared on the basis of the antibiotic
assay results. After 72 h of treatment, the wound bed was ready for placement of the
delivery system shown in Fig. 3, which was then protected with a sterile bandage.
The system was maintained unchanged on the wound for five days, when it was easily removed
without any pain to the patient, trauma to the zone, or damage to the graft.
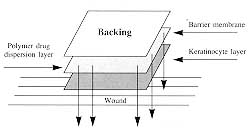
|
Fig.
3 - Mechanism of drug migration from a poylmeric dispersion, containing
antibiotics, through a modular dressing membrane combined with cultured keratinocytes. |
|
Results and discussion
Optimization of drug release from an
appropriate delivery system was obtained in order to evaluate the treatment of deep burns
with cultured keratinocytes combined with antibiotic therapy.
The antibiotic vancomycin, which was selected as the antibiotic model on the basis of
antibiotic assay results, possesses good water- solubility characteristics, while a
variety of commonly used topical formulations, including lipophilic ointments,
oil-in-water creams, and oily-type preparations, were not recommended. The administration
of simple aqueous sterile solutions would involve intermittent and often subtherapeutic
drug levels, a high frequency of application, and other serious obstacles.
To achieve the desired delivery rate we used a combined mechanism based on diffusion
through a membrane coupled with the decreased movement properties of the drug molecules in
the vehicle. The antibiotic was trapped in a water dispersion of hydroxyethylcellulose
used as a vehicle. This polymer is biocompatible, non-toxic, inexpensive, easily
available, and capable of forming gellike viscous dispersions. The amount of
hydroxyethylcellulose affects the viscosity of the formulation, which is one of the most
important parameters affecting the drug liberation rate owing to the reduced molecular
movement in the dispersion.
As the limiting step on drug transfer (i.e., the skin) is absent in burn patients, we
propose the use of a system in which the skin is replaced by a cultured keratinocyte layer
placed on a dressing membrane possessing specific permeability capacities.
The selection of the membrane was made on the basis of the observation of the diffusional
behaviour of commercially available materials commonly used in wound dressing: Adaptic@,
Trofoprocess@, TransprocessO, KeratoprocessO, and Veloderm@. Of these, Veloderm@ showed
the best diffusional characteristics. Permeation performance was assessed by detecting the
degree of movement and dispersal of coloration from a permanganate solution passing
through the membrane to the acceptor.
Fig. 3 presents a schematic representation of the drug release mechanism from the
proposed system.
The main components of the system were:
the cultured keratinocyte layer;
the biocompatible permeable membrane, which
acted as a physical support for the keratinocyte layer, as a rate-controlling diffusion
barrier, and as a dressing material to protect the wound and prevent penetration of
environmental agents;
the drug reservoir, consisting of the
hydrophilic drug incorporated in a suitable polymer gel-like sterile dispersion, used as a
vehicle, placed on the membrane in a layer about 5 mm thick;
the external backing, composed of
lipophilic occlusive petrolatum gauze, which occluded the system, prevented back diffusion
of hydrophilic drug molecules, and eliminated drug losses.
In vitro drug release experiments,
in which the skin was replaced by the permeable membrane, were performed in order to test
the behaviour of the formulation in conditions approaching in vivo conditions. Drug
release was followed by periodic measuring of the amount released in the simulated
acceptor fluid. The active ingredient leached from the system and transferred through the
membrane into the receptor phase was measured by UV spectrophotometric quantitative
analysis. The peaks observed were highly reproducible and were linearly related to
concentration over the range of 15 mg/100 ml. Drug flux was calculated by dividing the
total amount obtained by the transfer area and sampling interval.
Drug release was evaluated by plotting the percentage amount of drug discharged from the
formulation versus time. Fig. 2 shows the liberation profile of vancomycin.
The amounts of active ingredient released from the hydroxyethylcellulose dispersion showed
time dependence.
To establish the complete release time and the system's shelf life after application we
examined the potential mathematical correlation between drug amount released and time.
Since Higuchi's pioneering , studies various mathematical approaches based on differential
equations and thermodynamic and kinetic concepts of delivery have been proposed in order
to describe the release from topically applied drugs.` In previous publications" we
discussed the behaviour and main release models used to describe the drug discharged from
multiparticulate drug delivery systems.
We attempted to describe the release profile of vancomycin using a model function. On
application of differential rate treatments and linear regression analysis, the evidence
indicated that the amount of drug discharged increased linearly with the square root of
time, suggesting that the model for diffusion controlled transport should be followed. Fig.
4 shows the discharged drug amounts versus the square root of time. The
diffusion equation gave consistently higher values for the correlation coefficient
(0.996-0.999) than other equations (0.970-0.990).
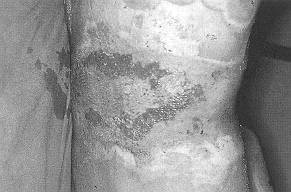 |
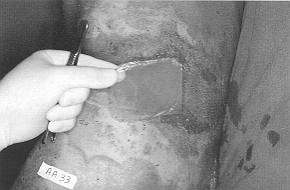 |
Figs. 4a, b - Clinical case: 3rd-degree burn in lumbar
region in which the autologous graft did not take owing to Staphylococcus infection. A, B
- positioning of autologous keratinocyte sheets mounted on petrolatum gauze (above) and on
Veloderm (below). |
|
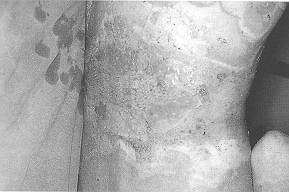 |
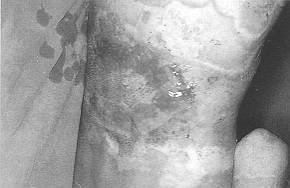 |
Fig. 4c - Positioning of autologous keratinocyte sheets
mounted on petrolatum gauze (above) and on Veloderm (below). |
Fig. 4d - Application of sheets on release system
containing specific antibiotic (vancomycin). |
|
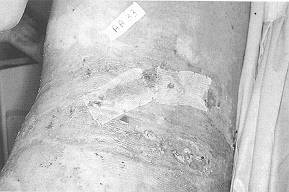 |
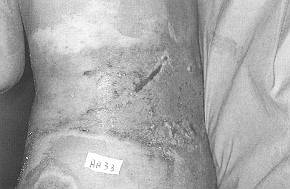 |
Fig. 4e - Medication on day 4 - comparison of sheets
mounted on petrolatum gauze and on Veloderm. |
Fig. 4f - Appearance on day 8. |
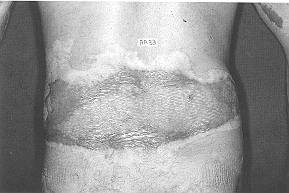 |
Fig.
4g - Final result. |
|
On the basis of these observations it was
possible, using geometric extrapolation, to predict that the drug administered by the
system would be sufficient for a therapy of 5-6 days with only one application. During the
clinical investigations it was observed that five days after a single application of the
antibiotic delivery system, when the bandage was removed for a check, the system was found
to have adhered perfectly to the treated surface. The whole system was then easily removed
and the wound area appeared dry and without any moist or serous exudate. This result was
probably a consequence of interactions between the exudate and the components of the
formulation applied, involving osmotic phenomena in the transfer through the membrane.
Eight days after application it was observed that the treatment of patients with the
cultured keratinocytes had been successful and that the lesions had healed.
Clinical cheeks showed no sign of sepsis, proving that the wounds had not been not
colonized by micro-organisms, which may cause inhibition of keratinocyte growth during the
period of application.
Conclusions
The topical release system limited the
indiscriminate use of broad-spectrum antibiotics, thus reducing the possible incidence of
undesirable multiresistance.
The modest dose of antibiotics administered produced constant drug levels in amounts
sufficient to inhibit sepsis and prolong action. Suspension of the system from the treated
area after five days was easy and caused no pain to the patient, no trauma to the zone,
and no damage to the graft. Eight days after application it was observed that treatment of
patients with the cultured keratinocytes had been successful and that the lesions had
healed.
RESUME. Les Auteurs
décrivent un nouveau système pour le transport des antibiotiques utilisable dans le
traitement des brûlures Le nouveau système a été conçu pour être employé avec
l'implantation des cellules-souches des kératinocytes cultivés épidermiques.
L'antibiotique a été bloqué dans un compartiment réservoir capable de libérer le
médicament à vélocité contrôlée. Le profil de transport désiré a été déterminé
in vitro en utilisant un modèle général linéaire à deux compartiments invariant dans
le temps. La vélocité appropriée pour le transport de l'antibiotique en quantité
suffisante pour une thérapie de 5-6 jours a été calculée moyennant un mécanisme
combiné basé sur la diffusion à travers une membrane et la diminution des propriétés
de mouvement du médicament du véhicule. Le système proposé pour la libération topique
a limité l'emploi sans discernement des antibiotiques à spectre large et réduit la
possibilité de la manifestation d'une multirésistance indésirable. L'interruption de
l'emploi du système dans la zone traitée après cinq jours n'a pas causé aucune douleur
pour les patients ni traumatismes localisés ni détérioration de la greffe. Les Auteurs
ont observé, huit jours après l'application, que le traitement des patients avec les
kératinocytes cultivés a été effectué avec succès et que les lésions se sont
cicatrisées.
BIBLIOGRAPHY
- Allgower M., Schoenenberger G.A., Sparkes B.G.: Burning the
largest immune organ. Burns, 21 (Suppl. 1): S7-S47, 1995.
- Odessey R.: Multicenter experience with cultured epidermal
autograft for treatment of burns. J. Burn Care Rehabil., 13: 174-80, 1992.
- Banakar U.V.: Percutancous absorption and principles of
drug delivery. In: "Transdermal drug product development", Banakar UN., Osborne
D.W. (eds), Technomic Publishing AG, 15-48, 1995.
- Rheinwald J.G., Green H.: Cell serial cultivation of
strains of human epidermal keratinocytes: the formation of keratinizing colonies from
single cells. Cell, 6: 331-44, 1975.
- Higuchi T.: Permeation of mechanical barriers by chemical
agents: Medical Laboratories Contract Report 32, Chemical Corp. Medical Laboratories, Army
Chem. Center, NM, 1954 and J. Pharm. Sci., 50: 874-5, 1961.
- Bunge A.L.: Predicting release rates from topical
formulations containing drugs in suspension. In: "Prediction of percutaneous
penetration, methods, measurements, modeling", vol. 2, Scott R.C., Guy R.H., Hadgraft
J., Bodd6 H.E. (eds), IBC Technical Services, 577-84, 1991.
- Giannola L.I., De Caro V., Rizzo M.C.: Preparation of white
beeswax microspheres loaded with valproic acid and kinetic study of drug release. Drug
Dev. Ind. Pharm., 21: 793-807, 1995.
- Giannola L.I., De Caro V., Severino A.: Camauba wax
microspheres loaded with valproic acid: preparation and evaluation of drug release. Drug
Dev. Ind. Pharm., 21: 1563-72, 1995.
- Giannola L.I., De Caro V.: Entrapment of phenytoin into
microspheres of oleaginous materials: process development and in vitro evaluation
of drug release. Drug Dev. Ind. Pharm., 23: 114552, 1997.
The authors are grateful to M.U.R.S.T. (Rome) for
its financial assistance.
This paper was received on 30 September 1998.Address correspondence to: Dr L.I. Giannola
Dipartimento di Chimica e Tecnologie Farmaceutiche
Università di Palermo, Palermo, Italy. |
|